Electrodes That Stimulate the Brain Reveal the Roots of Conscious Experience
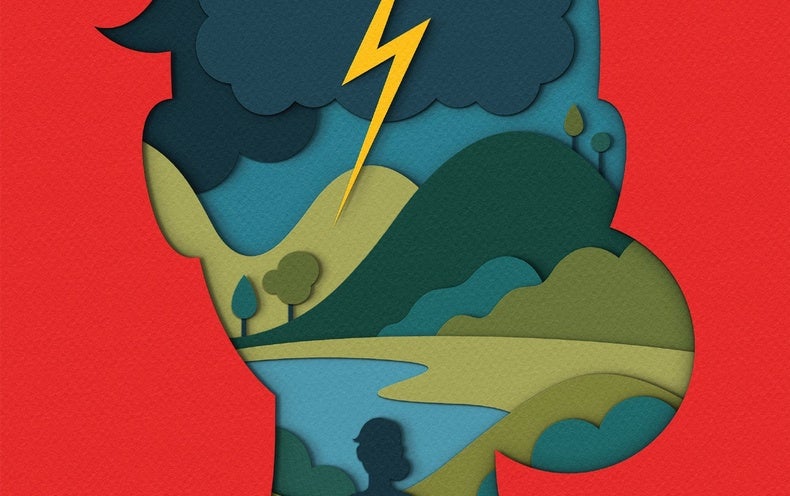
Consider the following experiences:
• You’re headed toward a storm that’s a couple of miles away, and you’ve got to get across a hill. You ask yourself: “How am I going to get over that, through that?”
• You see little white dots on a black background, as if looking up at the stars at night.
• You look down at yourself lying in bed from above but see only your legs and lower trunk.
These may seem like idiosyncratic events drawn from the vast universe of perceptions, sensations, memories, thoughts and dreams that make up our daily stream of consciousness. In fact, each one was evoked by directly stimulating the brain with an electrode. As American poet Walt Whitman intuited in his poem “I Sing the Body Electric,” these anecdotes illustrate the intimate relationship between the body and its animating soul. The brain and the conscious mind are as inexorably linked as the two sides of a coin.
Recent clinical studies have uncovered some of the laws and regularities of conscious activity, findings that have occasionally proved to be paradoxical. They show that brain areas involved in conscious perception have little to do with thinking, planning and other higher cognitive functions. Neuroengineers are now working to turn these insights into technologies to replace lost cognitive function and, in the more distant future, to enhance sensory, cognitive or memory capacities. For example, a recent brain-machine interface provides completely blind people with limited abilities to perceive light. These tools, however, also reveal the difficulties of fully restoring sight or hearing. They underline even more the snags that stand in the way of sci-fi-like enhancements that would enable access to the brain as if it were a computer storage drive.
Animal Electricity
Nervous systems operate on the flow of electric currents through ultradense and hyperconnected networks of switching elements. Countless physicians and scientists have worked on this problem over the past two and a half centuries, beginning with Italian physician Luigi Galvani, who in the late 18th century connected a freshly killed frog to a long metal wire. By pointing the wire toward the sky during a thunderstorm, he made the frog’s leg jump and twitch with each flash of lightning. Galvani’s investigations revealed that nerve fibers transmitted “animal electricity,” which is no different in kind from the “atmospheric electricity” that Benjamin Franklin discovered with his kite experiments in Philadelphia in 1752. In 1802 Galvani’s nephew Giovanni Aldini electrically stimulated the exposed brain of a decapitated prisoner during a public event. A jaw quivered. An eye opened. The spectacle may have helped to inspire Mary Shelley to write the classic gothic novel Frankenstein.
Subsequent animal studies demonstrated that exciting particular brain regions triggered movements in specific muscles and limbs. These investigations led to the discovery of the motor cortex in the 1870s. In 1874 American physician Robert Bartholow performed the first direct brain stimulation of a conscious patient—a pioneering act clouded in ethical controversy because it caused the patient pain and probably hastened her death. Intracranial electrical stimulation (iES) was refined over the following decades. It became part of the neurosurgeon’s toolbox thanks to the ground-breaking work of Wilder Penfield of the Montreal Neurological Institute, who between the 1930s and the 1950s used iES to map cortical areas that process motor or sensory functions.
In some people with epilepsy, drugs fail to adequately control the number or severity of seizures. Neurosurgery becomes an option if those seizures originate in a delimited neighborhood in the cortex—the outermost layer of the brain involved in perception, motor control, speech, reasoning, and so on—or in closely related structures, such as the hippocampus. Uncontrolled hyperexcitability starts because of local faulty wiring. It can grow and eventually engulf the rest of the brain. How much tissue to remove is a dilemma: cut too little, and seizures may continue; cut too much, and the patient may lose the ability to speak, see or walk. Surgeons must avoid areas of the cortex that are crucial for everyday behavior, such as the primary auditory, visual, somatosensory and motor cortices and the regions controlling understanding and producing speech, areas known as the eloquent cortex.
iES is brought in as a means to look for tissue that needs preserving. Neurosurgeons implant disk-shaped electrodes inside the skull, underneath the tough, leatherlike membrane known as the dura mater. Alternatively, they may insert needlelike electrodes into the brain’s gray matter to probe its function. Once the surgeons have identified the focal point of the seizure and removed the electrodes, they extract this tissue in a follow-up operation, and the patient usually becomes seizure-free.
A different use for iES is chronic electrical stimulation, in which the electrodes are left permanently in place. Gentle pulses of current sent through the electrodes can control the tremors and rigidity of Parkinson’s disease (a technique called deep-brain stimulation) or reduce the incidence and severity of seizures. Pilot clinical experiments are evaluating the use of such implanted electrodes as a visual prosthetic device to enable people with vision impairments to navigate and as a therapy for obsessive-compulsive disorder and depression.
Hot or Not
In July 2020 Nature Human Behaviour published an atlas highlighting locations across the cortex that, when aroused with electrodes, evoked conscious experiences, such as the storm and the disconnected body mentioned earlier. Led by Josef Parvizi, a professor of neurology at the Stanford University School of Medicine, the clinical team collected data from 67 people with epilepsy. The researchers recorded electrical activity from more than 1,500 sites in the cortex, primarily with subdural electrodes. They mapped the recordings from those sites to spots on a digital brain model so they could compare data from different brains (the pattern of ridges and valleys that give the organ the look of an oversized walnut differs from person to person). The team looked for “responsive” electrodes that triggered some visual or tactile sensation, muscle twitching or disrupted speech. If the patient did not feel anything when stimulated, that electrode was marked as nonresponsive.
Patients reported a range of electrode-evoked subjective experiences: briefly flashing points akin to stars of light; distorted faces like those in the paintings of Salvador Dalí; bodily feelings such as tingling, tickling, burning, pulsing and so-called out-of-body experiences; fear, unease, sexual arousal, merriment; the desire to move a limb; the will to persevere in the face of some great but unrecognized challenge. Mere tickling of neural tissue with a tiny bit of electric current was enough to evoke these feelings. During sham stimulation (no current applied), patients did not feel anything.
Although iES is safe and effective, it is also crude. The low-impedance electrodes are six to 10 square millimeters in area and deliver up to 10 milliamperes of electric current between adjacent electrodes—enough to modulate the excitability of a million or more nerve cells. Still, effects induced by iES can be quite localized. Responsiveness can change from all to none within millimeters or across a sulcus (a groove on the cortical surface).
The Parvizi team found that electrodes in the dedicated sensory and motor areas were far more likely to be responsive than those in areas of the cortex that process higher cognitive functions. Half to two thirds of electrodes above visual and tactile (somatosensory) cortex areas triggered some conscious perception; in regions of the lateral and anteromedial prefrontal cortex, which are involved with higher thought processes, at most one in five electrodes did so. Put differently, electrodes in the back of the cortex—in areas responsible for sensory experiences—were more likely to be active than those toward the front, which consists of regions of the cortex important for cognitive activity such as thinking, planning, moral reasoning, decision-making and intelligence.
Despite their importance for thinking, these regions have little to do with consciousness. Indeed, for the past century neurosurgeons have observed that so long as the eloquent cortex is spared, massive regions of the prefrontal cortex can be ablated without causing obvious deficits in the daily stream of consciousness of these patients. These regions of noneloquent cortex can modulate consciousness, but they are, by and large, not where conscious experience appears to originate. That privilege belongs to more posterior regions—the parietal, temporal and occipital lobes. Why the physical substrate of our mental experiences should be in the back rather than in the front of the brain remains a mystery.
To See or Not to See
Applying iES to the visual cortex triggers optical sensations known as phosphenes, brief flashes that resemble lightning striking a darkened plain. This observation is the source of a long-standing dream of a prosthetic device that restores some vision to people who are blind. Millions worldwide live with deficits in both eyes from retinitis pigmentosa, age-related macular degeneration, glaucoma, infection, cancer or trauma.
Doctors, scientists and engineers started pursuing visual prosthetics in the 1960s but have only recently been able to harness the appropriate technology to help blind people. One prominent example is a device known as Orion, developed by Second Sight Medical Products in Los Angeles. A tiny camera, mounted on glasses, converts images into pulses and transmits them wirelessly to fire 60 electrodes sitting on the visual cortex. The handful of people who have had this experimental device implanted into their brain perceive clouds of dots that allow them to navigate. “It’s still a blast every time I turn it on,” one study participant reports. “After seeing nothing to all of a sudden seeing little flickers of light move around and figuring out that they mean something. It’s just amazing to have some form of functional vision again.” Orion significantly improves the quality of life for people who previously lived in complete darkness. It enables them to safely cross the street or locate a doorway. But it does not allow them to regain the ability to recognize figures, shapes or letters.
A team at the University of California, Los Angeles, and the Baylor College of Medicine led by neurosurgeon Daniel Yoshor recently did accomplish this feat, as described in the journal Cell. They stimulated nearby locations in the visual cortex to trigger phosphenes that appear close together, demonstrating that the external visual environment is mapped in a regular fashion onto the surface of the visual cortex. This observation has led to the erroneous belief that individual phosphenes are like pixels on a computer display—that is, if you were to simultaneously stimulate a series of points on the cortical surface in the shape of a cross, the subject should see points forming a cross. This does not happen, however.
Stimulating more than one location yields unpredictable results. In one participant, simultaneous stimulation of five electrodes, each one associated with one discrete phosphene, triggered the illumination of two large phosphenes that did not coalesce into a letter or any other coherent form. If the researcher staggered activation of the electrodes in time, however, the subject could identify shapes. The staggering reflected the delay required to trace the shape of a letter, as if the researcher were outlining a letter into the hand of the subject or onto a piece of paper. In this more dynamic manner, the subject with the implant whose vision was blocked could identify a stimulus by tracing out a Z, N, V and W, rapidly distinguishing upward from downward motion or discriminating sequences of letters.
Seeing the shape of a single letter is not quite the same as seeing a glorious sunset over Homer’s wine-dark sea, but it represents progress. Why staggering stimulation in time improves perception is not clear and reveals our ignorance concerning functioning cortical circuits.
What Lies Ahead
Technological progress in so-called brain-machine interfaces is proceeding at a rapid pace. Elon Musk’s company Neuralink released in April of this year an impressive video showcasing a monkey playing the computer game Pong without any controller. This was achieved with two small chips implanted into the left and right motor cortices of the animal. Each chip has 1,024 hairlike electrodes that record the chattering of individual neurons. Collectively they convey the monkey’s intention to quickly move the paddle up or down the screen to return the ball to the opposite side. Everything was done wirelessly; no electronics or dangling wires were protruding from the monkey’s head. Many assume that surgeons will soon routinely replace or bypass faulty biological components—defective eyes or ears, failing memories—with superior electronic substitutes. Such optimism neglects the fact that all of this requires trepanation of the skull. In general, turning scientific insights into actual therapeutics is done in decades rather than in years. I am pretty confident that such enhancements will not occur in my lifetime (I’m now 64).
The “easiest” hurdles to overcome on the way to such a utopian (or perhaps dystopian) future are technological ones—reliably, quickly, and delicately reading and writing the brain electric. Neuralink’s device represents the best of currently available technology and will certainly improve in future iterations. But we still have a long way to go before we can identify which of the 50,000 or more neurons in any quinoa-sized bit of brain matter are involved in any given perception or action. Only when that happens will it be possible to limit electrical stimulation to just those neurons and not the output cables of nearby cells. That Parvizi and his colleagues failed to elicit conscious perceptions in more than half of all stimulated sites shows we lack tools capable of reliably eliciting any sensations through electrical stimulation, let alone being able to evoke any highly specific one.
Even more challenging are surgical and regulatory hurdles that demand that prosthetic devices can be routinely and safely implanted by drilling through the hard skull into the gray matter underneath while minimizing the risk of infections, bleeding and seizures. Furthermore, the electronics has to function for years inside warm, wet and salty biological tissue—hardly an optimal operating regime. You don’t want your prosthetic device to corrode or freeze up in the equivalent of the blue screen of death. For this reason, neural implants will remain a matter of last resort for those with severe sensory or motor impairments. As neuroprosthetic devices move through clinical trials, they will help people with visual impairments see and those with physical disabilities perform actions such as steering a wheelchair with their thoughts, like the mind-Pong-playing monkey. For everyone else, the benefits of highly invasive brain surgery are unlikely to outweigh the costs.
But the true Annapurna ahead involves understanding how three pounds of excitable brain matter is responsible for seeing, moving and suffering. Yes, the physical substrate of heaven and hell is rooted in bioelectric signals that obey natural laws. But that tells us precious little about how a trillion electrical signals spiking each second, streaming over networks of tens of billions of heterogeneous cells, constitute a sight, sound or emotion.
Intracranial brain stimulation highlights the daily miracle of the brain’s water changing into the wine of consciousness. The question remains, though: What is it about the brain, the most complex piece of active matter in the known universe, that turns the activity of 86 billion neurons into the feeling of life itself?
World News || Latest News || U.S. News
Source link